Ever since the dawn of the Space Age with the launch of Sputnik 1 in 1957, humanity has been captivated by the prospect of exploring the cosmos. The subsequent years witnessed remarkable achievements, such as Yuri Gagarin becoming the first human in space in 1961, the Apollo 11 Moon landing in 1969, and the ongoing presence of humans on the International Space Station since 2000. These milestones, along with the advancements in space probes, telescopes, and satellites, have not only expanded our understanding of the universe but also fueled our collective imagination.
Importance of Speed in Space Exploration
As we continue to reach farther into the cosmos, the speed at which we can travel in space becomes increasingly significant. The vast distances between celestial bodies make traditional methods of propulsion, like chemical rockets, insufficient for timely exploration. In order to visit neighboring star systems or even more remote regions of our galaxy, we must develop faster means of transportation that can overcome the limitations of time and distance.
- Higher speeds in space travel offer numerous advantages. For manned missions, faster travel times reduce the duration of exposure to harmful radiation and microgravity, thereby mitigating the negative effects on astronauts’ health.
- For unmanned missions, increased speeds enable us to study more celestial objects within a given timeframe, thereby accelerating our understanding of the universe.
- Furthermore, faster space travel can increase the likelihood of discovering potential habitable worlds and extraterrestrial life forms, ultimately broadening our understanding of life’s origins and the potential for future human settlements.
In this article, we will delve into the current state of space travel technology, examining the fastest propulsion methods available today and the limitations that define our cosmic speed boundaries. Additionally, we will explore cutting-edge research and development in propulsion technology that may pave the way for rapid advancements in space exploration in the near future.
How Fast Can We Travel in Space with Current Technology
The Speed of Light: The Ultimate Limit
The speed of light, denoted as “c,” is a fundamental constant of nature that plays a critical role in our understanding of the universe. It is approximately 299,792 kilometers per second (km/s) or about 186,282 miles per second (mi/s) in a vacuum. According to Albert Einstein’s Special Theory of Relativity, nothing can travel faster than the speed of light, making it the ultimate speed limit for any object in the universe.
This cosmic speed limit has profound implications for space travel. At the speed of light, it would still take over four years to reach the nearest star to our solar system, Proxima Centauri, which is 4.24 light-years away. For perspective, it would take about 6,300 years to cover the same distance using the fastest spacecraft ever launched, the Parker Solar Probe, which reached speeds of up to 700,000 km/h (430,000 mph) during its mission.
Time Dilation and Its Effects on Space Travel
As an object approaches the speed of light, it experiences a phenomenon known as time dilation. Time dilation is a consequence of the Special Theory of Relativity, which states that time appears to slow down for an object in motion relative to an observer at rest. The faster the object moves, the more pronounced the time dilation effect becomes.
For space travel, this means that as a spacecraft’s speed increases, the flow of time onboard the spacecraft slows down compared to the flow of time for an observer on Earth. For example, if a spacecraft were to travel at a speed close to the speed of light, a journey that lasts a few years for the astronauts onboard might equate to several decades or even centuries for observers back on Earth.
Time dilation introduces unique challenges for interstellar missions, particularly manned ones. While astronauts traveling at relativistic speeds might age more slowly than their counterparts on Earth, they would return to a world that has aged significantly more than they have. This effect poses challenges not only for the psychological and emotional well-being of astronauts but also for the planning and execution of long-duration space missions.
Chemical Propulsion: The Traditional Method
Chemical propulsion has been the backbone of space travel since its inception. It relies on the combustion of chemical propellants to produce thrust, propelling a spacecraft forward. Chemical rockets, such as the Saturn V that carried the Apollo astronauts to the Moon and the Space Shuttle’s main engines, have demonstrated impressive capabilities in lifting heavy payloads and enabling human spaceflight.
However, chemical propulsion has significant limitations.
- First, the propellant mass required to generate thrust contributes to a large portion of a rocket’s total mass, making it less efficient for long-distance travel. The Tsiolkovsky rocket equation demonstrates this challenge, highlighting that the required fuel increases exponentially as the desired change in velocity (delta-v) increases. Consequently, a large amount of fuel is needed to achieve high speeds, which in turn increases the spacecraft’s mass and demands even more fuel.
- Second, chemical rockets have a relatively low specific impulse, a measure of propulsion system efficiency. The specific impulse of chemical rockets is limited by the energy content of the chemical propellants and the temperature constraints of the combustion process. This limitation translates to lower achievable speeds compared to other propulsion methods, such as electric or nuclear propulsion.
Achievable Speeds with Chemical Propulsion
The speeds achievable with chemical propulsion depend on the type of rocket, the propellant used, and the mission profile. Generally, chemical rockets can reach speeds of up to 40,000 km/h (25,000 mph) or more. For example, the New Horizons probe, which was launched in 2006 to study Pluto and the Kuiper Belt, achieved a speed of about 58,000 km/h (36,000 mph) using chemical propulsion.
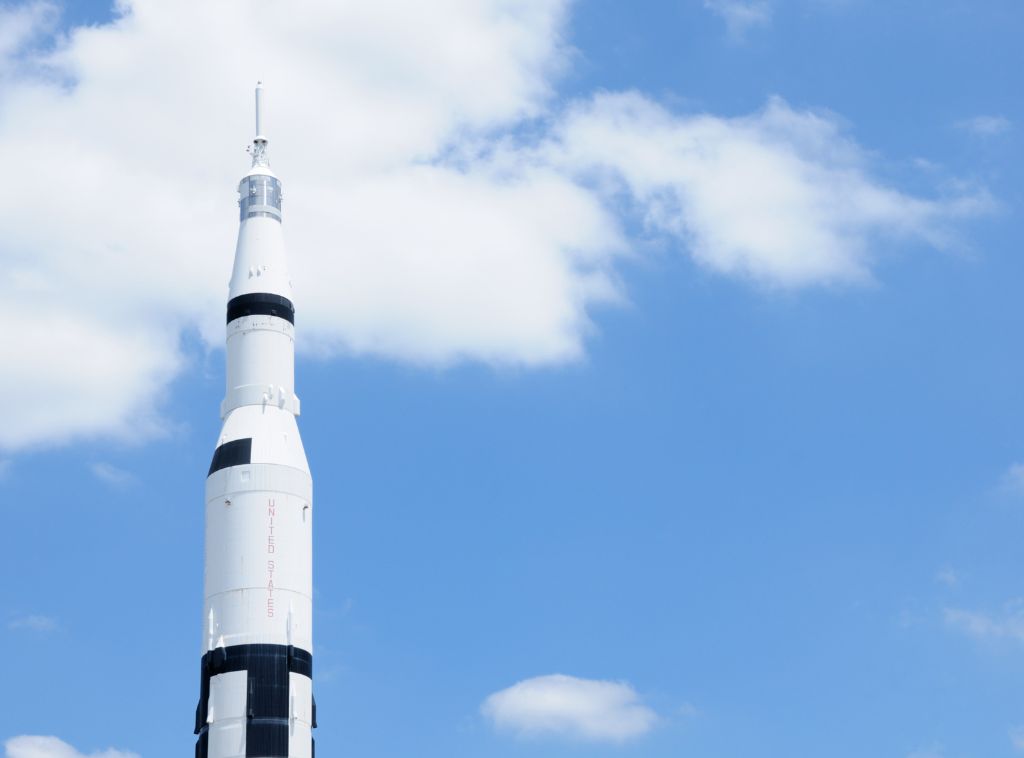
Iconic Missions Using Chemical Rockets
Many iconic missions in space exploration have relied on chemical propulsion.
- The Apollo program, which landed astronauts on the Moon between 1969 and 1972, used the Saturn V rocket, a chemical rocket that remains the tallest, heaviest, and most powerful rocket ever flown.
- Another well-known example is the Space Shuttle, which operated from 1981 to 2011, using a combination of solid rocket boosters and liquid-fueled main engines for its propulsion.
While chemical propulsion has been instrumental in many groundbreaking space missions, its limitations in efficiency and achievable speeds highlight the need for alternative propulsion methods to enable more ambitious space exploration endeavors.
Ion Propulsion: The Electric Alternative
Ion propulsion, also known as electric propulsion, offers a promising alternative to traditional chemical propulsion systems. Ion thrusters work by ionizing a neutral gas, typically xenon or argon, and then accelerating the charged particles (ions) through an electric field to produce thrust. Since ion thrusters do not rely on combustion, they can achieve much higher specific impulse compared to chemical rockets, making them more fuel-efficient and capable of achieving higher speeds over long durations.
Advantages and Limitations of Ion Propulsion
Ion propulsion offers several advantages over chemical propulsion:
- Higher specific impulse: Ion thrusters have a much higher specific impulse, meaning they can generate more thrust per unit of propellant mass. This results in significant fuel savings and allows spacecraft to carry more scientific instruments or cargo.
- Long operational lifetimes: Ion thrusters can operate continuously for years, enabling spacecraft to maintain thrust for extended periods and achieve higher speeds over time.
- Reduced mass: Due to their fuel efficiency, ion propulsion systems can substantially reduce the overall mass of a spacecraft, leading to cost savings during launch and increased mission flexibility.
However, ion propulsion also has some limitations:
- Low thrust: Ion thrusters produce relatively low levels of thrust compared to chemical rockets. This makes them unsuitable for time-sensitive missions or those requiring high acceleration, such as launching a spacecraft from Earth’s surface.
- Power requirements: Ion propulsion systems require a significant amount of electrical power, typically provided by solar panels or nuclear reactors. This can add complexity and weight to a spacecraft’s design.
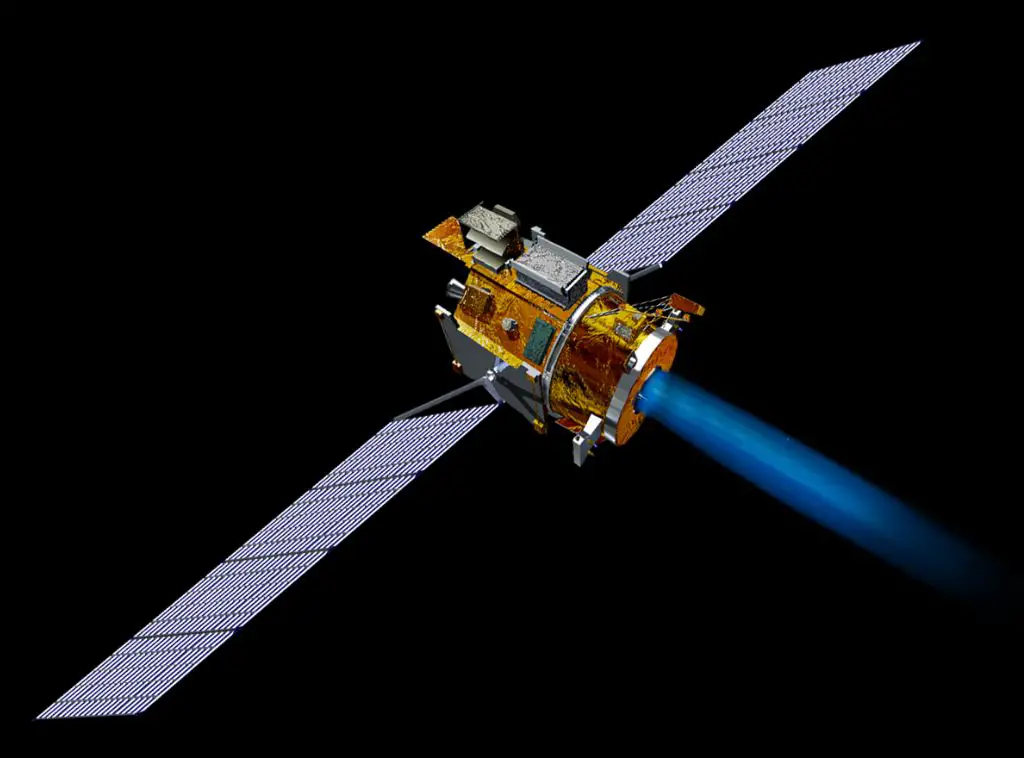
Successful Missions Using Ion Propulsion
Ion propulsion has been successfully employed in several space missions, showcasing its potential for deep space exploration:
- Deep Space 1: Launched in 1998, NASA’s Deep Space 1 was the first mission to use ion propulsion as its primary propulsion system. The spacecraft tested several advanced technologies, including the NSTAR ion thruster, which propelled it to a successful flyby of asteroid 9969 Braille and comet Borrelly.
- Dawn: The Dawn mission, launched in 2007, used ion propulsion to visit and study two large objects in the asteroid belt, Vesta and Ceres. The spacecraft’s ion thrusters allowed it to enter and exit orbits around both celestial bodies, a feat that would have been impossible with conventional chemical propulsion due to the amount of fuel required.
- Hayabusa and Hayabusa2: The Japan Aerospace Exploration Agency (JAXA) employed ion propulsion for its Hayabusa missions, which aimed to collect and return samples from asteroids. Hayabusa, launched in 2003, successfully returned samples from asteroid 25143 Itokawa in 2010. Hayabusa2, launched in 2014, collected samples from asteroid 162173 Ryugu and returned them to Earth in 2020.
Solar Sails: Harnessing the Power of the Sun
Solar sails represent an innovative propulsion method that utilizes the power of sunlight to propel spacecraft. These sails are made of lightweight, highly reflective materials, such as Mylar or Kapton, that capture the momentum of photons emitted by the Sun. As photons strike the sail, they transfer their momentum to the spacecraft, generating a small but continuous force that propels it forward. Unlike chemical or ion propulsion, solar sails require no propellant, making them an attractive option for long-duration missions.
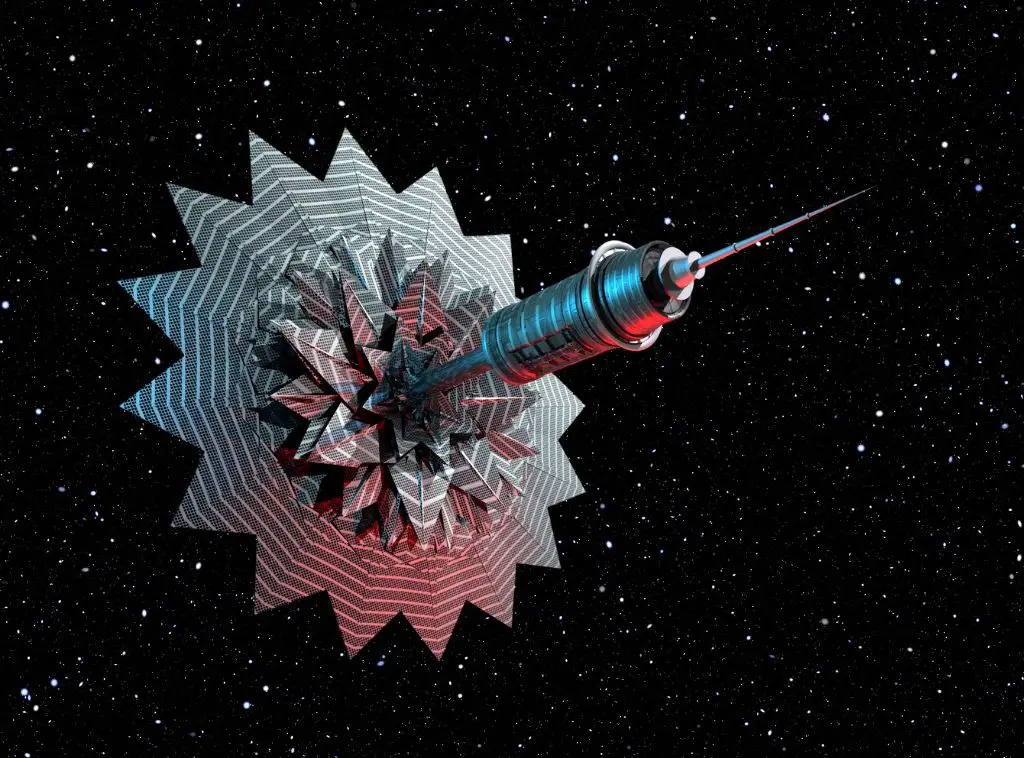
Achievable Speeds and Limitations of Solar Sail Technology
Solar sails can achieve significant speeds over time due to the continuous and propellant-free nature of their propulsion. Theoretically, solar sails could reach speeds of up to hundreds of thousands of kilometers per hour, depending on the sail’s size, mass, and distance from the Sun. However, the actual speeds achieved will depend on various factors, including the sail’s design, the spacecraft’s mass, and mission constraints.
Despite their potential, solar sails also have limitations:
- Diminishing thrust with distance: As a spacecraft travels farther from the Sun, the intensity of sunlight decreases, reducing the thrust generated by the solar sail. This makes solar sails less effective for missions to the outer solar system and beyond.
- Low initial thrust: Solar sails generate relatively low thrust, especially when compared to chemical rockets. This means they are not suitable for time-sensitive missions or those requiring rapid acceleration.
- Deployment and control challenges: Designing, deploying, and controlling large, lightweight solar sails present significant engineering challenges,as they need to be carefully unfolded in space and controlled to maintain the correct orientation relative to the Sun. Additionally, space debris and micrometeoroids pose a risk to the sail’s delicate structure, potentially compromising its performance.
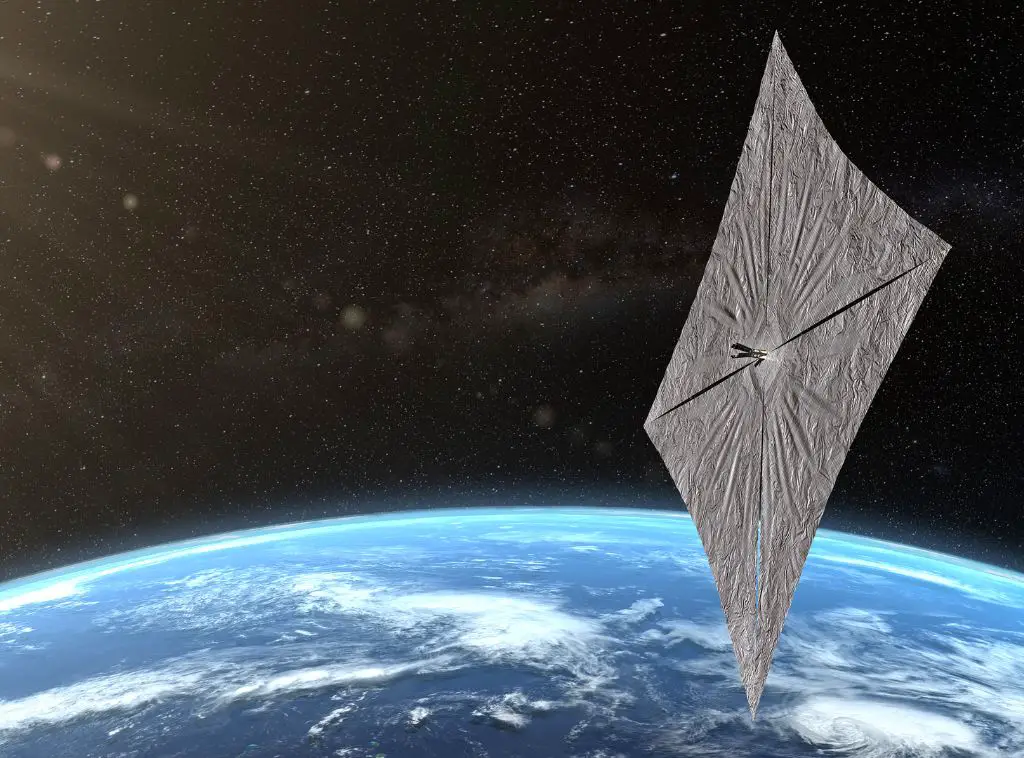
Notable Solar Sail Missions
Several solar sail missions have been launched to demonstrate the viability and potential of this propulsion method:
IKAROS: Launched in 2010 by the Japan Aerospace Exploration Agency (JAXA), IKAROS (Interplanetary Kite-craft Accelerated by Radiation Of the Sun) was the first spacecraft to successfully demonstrate solar sail propulsion in interplanetary space. The spacecraft used a 14-meter (46 ft) diagonal square sail to propel itself during its mission to Venus and beyond.
LightSail: Developed by The Planetary Society, the LightSail project aimed to demonstrate controlled solar sail propulsion in Earth orbit. LightSail 1, launched in 2015, was a proof-of-concept mission that successfully deployed its solar sail but did not achieve controlled propulsion. LightSail 2, launched in 2019, successfully deployed its solar sail and demonstrated controlled solar sailing, adjusting its orbit using only the pressure of sunlight.
These missions highlight the potential of solar sail technology for propellant-free propulsion in space. With continued research and development, solar sails could play an increasingly important role in future space exploration, enabling missions to distant celestial bodies and even interstellar travel.
Nuclear Propulsion: The Untapped Potential
Nuclear propulsion offers a promising alternative to traditional propulsion methods for long-distance space travel. There are two primary concepts of nuclear propulsion: nuclear thermal propulsion (NTP) and nuclear electric propulsion (NEP).
Nuclear Thermal Propulsion (NTP):
NTP systems use a nuclear reactor to heat a propellant, typically hydrogen, to high temperatures. The heated propellant is then expelled through a nozzle to generate thrust. NTP offers a higher specific impulse than chemical propulsion systems and can provide greater thrust than electric propulsion systems, making it an attractive option for crewed missions to Mars and beyond.
Nuclear Electric Propulsion (NEP):
NEP systems use a nuclear reactor to generate electricity, which is then used to power electric propulsion systems, such as ion or Hall-effect thrusters. NEP offers a higher specific impulse than NTP systems but typically generates lower thrust. This makes NEP well-suited for long-duration, uncrewed missions with lower acceleration requirements.
Speed Capabilities and Advantages of Nuclear Propulsion
Nuclear propulsion offers several advantages over traditional propulsion methods, including increased speed capabilities:
- Higher specific impulse: Both NTP and NEP systems have a higher specific impulse than chemical propulsion, resulting in increased fuel efficiency and reduced mass requirements for long-duration missions.
- Greater thrust: NTP systems can generate higher thrust levels than ion propulsion systems, enabling faster transit times for crewed missions.
- Continuous operation: Nuclear reactors can operate continuously for years, allowing spacecraft to maintain thrust for extended periods and achieve higher speeds over time. This makes nuclear propulsion particularly suitable for deep space missions, where the constant acceleration can reduce travel times significantly compared to conventional propulsion systems.
- Scalability: Nuclear propulsion systems can be scaled up to accommodate larger spacecraft and payloads, providing a viable option for ambitious missions that require significant power and propulsion capabilities.
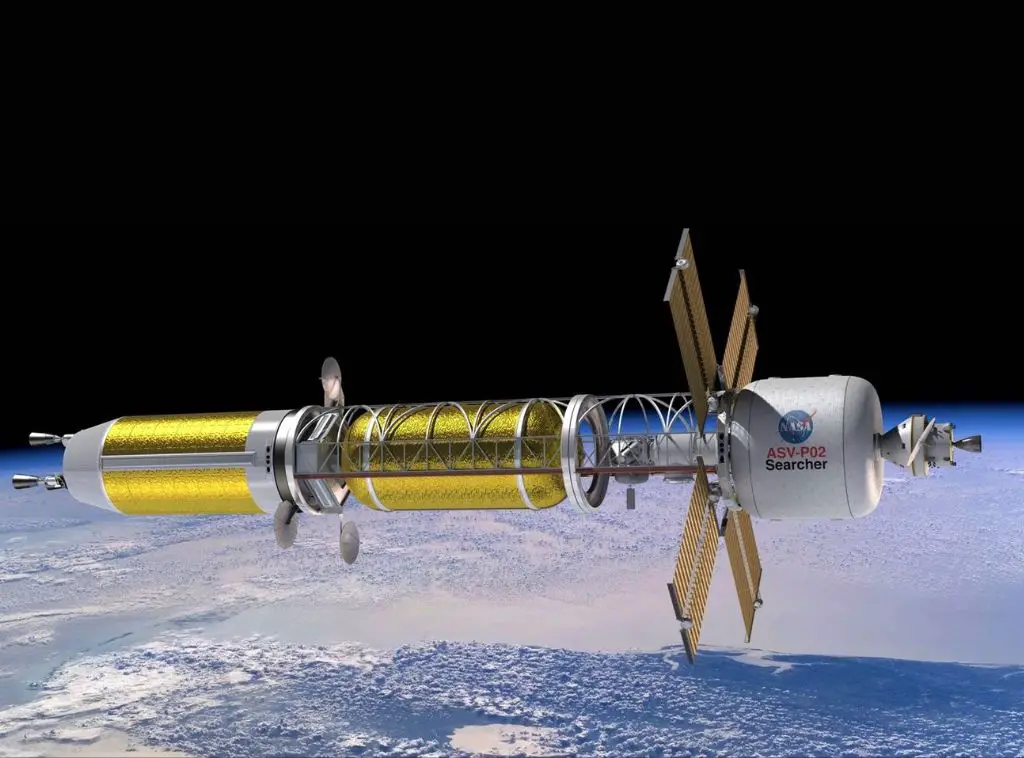
Current Research and Development in Nuclear Propulsion Technology
There is ongoing research and development aimed at advancing nuclear propulsion technology and overcoming the challenges associated with its implementation:
- NASA’s Space Nuclear Propulsion Project: In collaboration with the U.S. Department of Energy, NASA is working on the development of nuclear propulsion systems for potential crewed missions to Mars. The project focuses on demonstrating the feasibility, safety, and performance of NTP systems, with the goal of enabling faster transit times and increased mission flexibility.
- DARPA’s DRACO Program: The Defense Advanced Research Projects Agency (DARPA) has initiated the DRACO (Demonstration Rocket for Agile Cislunar Operations) program, which aims to develop and demonstrate a high-assay, low-enriched uranium (HALEU) nuclear thermal propulsion system. The program seeks to enable rapid maneuverability for spacecraft in cislunar space, enhancing the United States’ capabilities in this strategic domain.
- ROSATOM’s Nuclear Electric Propulsion Project: Russia’s state atomic energy corporation, ROSATOM, is working on a nuclear electric propulsion system for deep space missions. The project aims to develop a megawatt-class space nuclear power plant that could power high-efficiency electric propulsion systems, potentially enabling faster and more efficient interplanetary missions.
Breakthrough Propulsion Technologies: A Glimpse into the Future
While current propulsion technologies have allowed us to explore our solar system, more advanced concepts are being studied to enable even faster and farther-reaching space travel. Some of these futuristic propulsion methods include antimatter propulsion and warp drive, which are still in the theoretical and experimental stages.
- Antimatter Propulsion: Antimatter propulsion systems rely on the immense energy released when matter and antimatter particles come into contact and annihilate each other. In theory, this could provide an extremely efficient propulsion method, with specific impulses far beyond those achievable with chemical, electric, or nuclear propulsion. However, producing, storing, and controlling antimatter remain significant challenges that need to be overcome before such a propulsion system could become a reality.
- Warp Drive: Inspired by the concept of faster-than-light travel popularized in science fiction, warp drive envisions a method of space travel that involves bending spacetime around a spacecraft, allowing it to effectively “jump” vast distances instantaneously. While the idea is based on the principles of general relativity, there are currently no known methods to create the required spacetime distortions, and the concept remains purely theoretical at this stage.
Theoretical Speeds Achievable with Breakthrough Technologies
The speeds achievable with breakthrough propulsion technologies, if realized, could be orders of magnitude higher than those possible with current methods:
- Antimatter Propulsion: The energy released during matter-antimatter annihilation is extremely high, potentially enabling speeds approaching a significant fraction of the speed of light. This would allow for interstellar travel within human timescales, making it possible to explore nearby star systems.
- Warp Drive: If a feasible method for creating and controlling spacetime distortions were discovered, warp drive could, in theory, enable faster-than-light travel, revolutionizing our understanding of space exploration and potentially opening up the entire galaxy for human exploration.
Current Research and Development Status
Although these advanced propulsion concepts hold tremendous promise, they are still in the early stages of research and development:
- Antimatter Propulsion: While some preliminary experiments have been conducted on producing and storing antimatter, the quantities produced are minuscule, and the technology for controlling and utilizing antimatter for propulsion is still in its infancy. Research in this area is ongoing, but practical antimatter propulsion systems remain a distant possibility.
- Warp Drive: Current research on warp drive is primarily theoretical, focusing on finding solutions to the complex problems associated with bending spacetime. Experimental verification of these ideas is still far beyond our current technological capabilities, and it is unclear if warp drive can ever become a practical means of propulsion.
While breakthrough propulsion technologies offer tantalizing glimpses into the future of space travel, significant scientific and engineering challenges must be overcome before they can be realized. In the meantime, the ongoing development of existing propulsion methods, such as ion, solar sail, and nuclear propulsion, will continue to push the boundaries of human space exploration.
In conclusion, the quest for faster and more efficient space travel remains an ongoing endeavor that continues to captivate scientists, engineers, and visionaries alike. Current propulsion technologies, such as chemical, ion, solar sail, and nuclear propulsion, have opened up our solar system to exploration and will continue to improve as research and development progress. Meanwhile, breakthrough propulsion concepts, like antimatter propulsion and warp drive, offer tantalizing glimpses into a future where interstellar travel could become a reality. As our understanding of the universe expands and our technological capabilities advance, the potential for faster and farther-reaching space travel will continue to push the boundaries of human exploration and our pursuit of new frontiers in the cosmos.